History of physics
2008/9 Schools Wikipedia Selection. Related subjects: History; Physics
History of science |
Background |
---|
Theories/sociology |
Historiography |
Pseudoscience |
By era |
In early cultures |
in Classical Antiquity |
In the Middle Ages |
In the Renaissance |
Scientific Revolution |
By topic |
Natural sciences |
Astronomy |
Biology |
Chemistry |
Ecology |
Geography |
Geology |
Paleontology |
Physics |
Social sciences |
Economics |
Linguistics |
Political science |
Psychology |
Sociology |
Technology |
Agricultural science |
Computer science |
Materials science |
Medicine |
Navigational pages |
Timelines |
Portal |
Categories |
Since antiquity, human beings have sought to understand the workings of nature: why unsupported objects drop to the ground, why different materials have different properties, the character of the universe such as the form of the Earth and the behaviour of celestial objects such as the Sun and the Moon, and so forth. Typically the behaviour and nature of the world was explained by invoking the actions of gods. Eventually explanations were proposed based on philosophical speculation. Rarely verified by systematic experimental testing, many of them were wrong, but this is part of the dialectical nature of scientific inquiry, and even modern theories of quantum mechanics and relativity are merely considered "theories that have not been broken yet".
The growth of physics has brought not only fundamental changes in ideas about the material world, mathematics and philosophy, but also transformed society, through the development of technology by the engineering profession. Physics is considered both a body of knowledge and the practice that makes and transmits it. Experimental physics began in the Middle Ages with experimental investigations into optics, statics and dynamics. The Scientific Revolution, beginning around 1543, is considered a convenient boundary between ancient thought and classical mechanics. The emergence of physics as a science distinct from natural philosophy began with the Scientific Revolution of the 16th and 17th centuries, and continued through the dawn of modern physics in the early 20th century. The year 1900 marks the beginnings of a more modern physics. Today, the science shows no sign of completion, as more issues are raised, with questions rising from the age of the universe, to the nature of the vacuum, to the ultimate nature of the properties of subatomic particles. Partial theories are currently the best that physics has to offer. The list of unsolved problems in physics is large.
Antiquity
The first records of the recognition that astronomical phenomena are periodic and of the application of mathematics to their prediction is Babylonian. Tablets dating back to the Old Babylonian period ( 2nd millennium BC) document the application of mathematics to the variation in the length of daylight over a solar year. Centuries of Babylonian observations of celestial phenomena are recorded in the series of cuneiform tablets known as the Enūma Anu Enlil.. Babylonian astronomy was the basis for much of what was done in Greece, in India, in Sassanian Iran, in Byzantium, in Syria, in Islam, in Central Asia, and in Western Europe.
Further investigations into early ideas in physics began with eminent Greek pre-Socratic philosophers such as Thales, Anaximander, possibly Pythagoras, Heraclitus, Anaxagoras, Empedocles and Philolaus, many of whom were involved in various schools. For example, Anaximander and Thales belonged to the Milesian school.
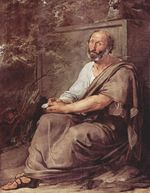
Plato, briefly and Aristotle at length, continued these studies of nature in their works, the earliest surviving complete treatises dealing with natural philosophy. Democritus, a contemporary, was of the school of Atomists who attempted to characterize the nature of matter. Similar atomic philosophy would develop in ancient India. Due to the absence of advanced experimental equipment such as telescopes and accurate time-keeping devices, experimental testing of physical hypotheses was impossible or impractical. There were exceptions and there are anachronisms. Greek thinkers like Archimedes proposed calculating the volume of objects like spheres and cones by dividing them into very thin disks and adding up the volume of each disk, using methods resembling integral calculus. It was also Archimedes who derived many correct quantitative descriptions of mechanics and also hydrostatics when, so the story goes, he noticed that his own body displaced a volume of water while he was getting into a bath one day. In doing so Archimedes would be the first to uncover a law of nature. Another remarkable example was that of Eratosthenes, who deduced that the Earth was a sphere, and accurately calculated its circumference using the shadows of vertical sticks to measure the angle between two widely separated points on the Earth's surface.
Modern knowledge of many early ideas in physics, and the extent to which they were experimentally tested, is unknown. Almost all direct record of these ideas was lost when the Library of Alexandria was destroyed, around 400 AD. Perhaps the most remarkable idea we know of from this era was the deduction by Aristarchus of Samos that the Earth was a planet that traveled around the Sun once a year, and rotated on its axis once a day (accounting for the seasons and the cycle of day and night), and that the stars were other, very distant suns which also had their own accompanying planets (and possibly, lifeforms upon those planets).
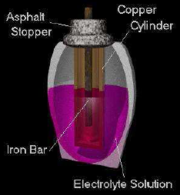
The discovery of the Antikythera mechanism, which is considered to be the earliest analog computer, points to a detailed understanding of movements of these astronomical objects, as well as a use of gear-trains that pre-dates any other known civilization's use of gears, except that of ancient China. The discovery of the Baghdad Battery also suggests that a primitive form of electricity may have been known in Mesopotamia during the Parthian or Sassanid periods.
A primitive steam-powered device, Hero's aeolipile, was only a curiosity which did not solve the problem of transforming its rotational energy into a more usable form, not even by gears. The Archimedes screw is still in use today, to lift water from rivers onto irrigated farmland. The simple machines were unremarked, with the exception (at least) of Archimedes' elegant proof of the law of the lever. Ramps were in use several millennia before Archimedes, to build the Pyramids.
A particularly important ancient contribution that would allow physics to develop into a science came from India. It was the introduction of the Hindu-Arabic numerals. Modern physics can hardly be imagined without a system of arithmetic in which simple calculation is easy enough to make large calculations even possible. The modern positional numeral system (the Hindu-Arabic numeral system) and the number zero were first developed in India.
Physics in the Middle Ages
Islamic world
Like the later Scientific revolution in the West, Islamic science was built on a foundation laid in antiquity, in this case, the intellectual patrimony of the Byzantines, Persians and Indians they conquered. The Arab and Persian scholars of the Islamic Golden Age made advances by building on previous work in astronomy, mathematics, and physics while developing new fields like modern optics, experimental physics, and hydrodynamics.
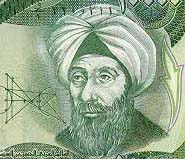
The most important scientific development during the Middle Ages was the pioneering development of the experimental scientific method by Ibn al-Haytham (commonly Latinized Alhazen, ca. 965–1040), as recorded in his Book of Optics. Alhazen, who is regarded as the "father of modern optics", and a pioneer of the scientific method and experimental physics, developed a broad theory that explained vision, using geometry and anatomy, which stated that each point on an illuminated area or object radiates light rays in every direction, but that only one ray from each point, which strikes the eye perpendicularly, can be seen. The other rays strike at different angles and are not seen. He built a camera obscura and used the example of the pinhole camera, which produces an inverted image, to support his argument. This contradicted Ptolemy's emission theory of vision that objects are seen by rays of light emanating from the eyes.
Alhazen held light rays to be streams of minute particles travelling at a finite speed. He improved Ptolemy's theory of the refraction of light, and went on to discover the law of refraction. He also carried out the first experiments on the dispersion of light into its constituent colors. His major work Book of Optics was translated into Latin in the Middle Ages, as well as his book dealing with the colors of sunset. He dealt at length with the theory of various physical phenomena like shadows, eclipses, and the rainbow. He also attempted to explain binocular vision and the moon illusion. Through these extensive researches on optics, he is considered the "father of modern optics". Alhazen also correctly argued that we see objects because the sun's rays of light, which he believed to be streams of tiny particles traveling in straight lines, are reflected from objects into our eyes. He understood that light must travel at a large but finite velocity, and that refraction is caused by the velocity being different in different substances. He also studied spherical and parabolic mirrors, and understood how refraction by a lens will allow images to be focused and magnification to take place. He understood mathematically why a spherical mirror produces spherical aberration.
Avicenna (980-1037) agreed that the speed of light is finite, as he "observed that if the perception of light is due to the emission of some sort of particles by a luminous source, the speed of light must be finite." Abū Rayhān al-Bīrūnī (973-1048) also agreed that light has a finite speed, and he was the first to discover that the speed of light is much faster than the speed of sound. Qutb al-Din al-Shirazi (1236-1311) and Kamāl al-Dīn al-Fārisī (1260-1320) gave the first correct explanations for the rainbow phenomenon.
In mechanics, the eldest Banū Mūsā brother, Muhammad ibn Musa, in his Astral Motion and The Force of Attraction, hypothesized that there was a force of attraction between heavenly bodies in the 9th century. Abū Rayhān al-Bīrūnī (973-1048), and later al-Khazini, developed experimental scientific methods for mechanics, especially the fields of statics and dynamics, particularly for determining specific weights, such as those based on the theory of balances and weighing. Muslim physicists unified statics and dynamics into the science of mechanics, and they combined the fields of hydrostatics with dynamics to give birth to hydrodynamics. They applied the mathematical theories of ratios and infinitesimal techniques, and introduced algebraic and fine calculation techniques into the field of statics. They were also generalized the theory of the centre of gravity and applied it to three-dimensional bodies. They also founded the theory of the ponderable lever and created the "science of gravity" which was later further developed in medieval Europe. Al-Biruni also theorized that acceleration is connected with non-uniform motion.
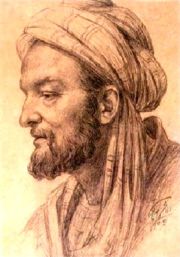
In the early 11th century, Ibn al-Haytham discussed the theory of attraction between masses, and it seems that he was aware of the magnitude of acceleration due to gravity, and he stated that the heavenly bodies "were accountable to the laws of physics". also enunciated the law of inertia when he stated that a body moves perpetually unless an external force stops it or changes its direction of motion. He also developed the concept of momentum, though he did not quantify this concept mathematically. His contemporary Avicenna also developed the concept of momentum, referring to impetus as being proportional to weight times velocity, for which he is considered a pioneer of the concept of momentum. His theory of motion was also consistent with the concept of inertia in classical mechanics.
In 1121, al-Khazini, in his treatise The Book of the Balance of Wisdom, further elaborated an idea proposed by the Greeks that all bodies are attracted towards the centre of the earth. Al-Khazini also developed the concept of gravitational potential energy and was one of the first to clearly differentiate between force, mass, and weight. Avempace (d. 1138) argued that there is always a reaction force for every force exerted, though he did not refer to the reaction force as being equal to the exerted force. His theory of motion had an influence on later physicists like Galileo Galilei. His contemporary Hibat Allah Abu'l-Barakat al-Baghdaadi was the first to negate Aristotle's idea that a constant force produces uniform motion, and he instead argued that a force applied continuously produces acceleration, an important concept in classical mechanics. He also described acceleration as the rate of change of velocity. Averroes (1126–1198) defined and measured force as "the rate at which work is done in changing the kinetic condition of a material body" and correctly argued "that the effect and measure of force is change in the kinetic condition of a materially resistant mass." In the early 16th century, al-Birjandi developed a hypothesis similar to "circular inertia." The Muslim developments in mechanics laid the foundations for the later development of classical mechanics in early modern Europe.
Medieval Europe
Following the collapse of the Western Roman Empire, the knowledge of classical antiquity was preserved in its monasteries, in the Byzantine Empire, and in the Islamic world. Works lost in Western Christendom but preserved in the Islamic world led clerical scholars such as Michael the Scot to learn Arabic in order to study them. Their translations made available to medieval Europe not only the works of the ancients, but also contemporary work. Works both ancient and contemporary also became known in medieval Europe through such points of contact as the Republic of Venice, al-Andalus, and returning Crusaders. By providing a locus for the exchange of ideas and scholarly collaboration, the birth of the medieval university was key to the intellectual revitalization of Europe.
By the 13th century, precursors of the modern scientific method can be seen on Robert Grosseteste's emphasis on mathematics as a way to understand nature and on the empirical approach admired by Roger Bacon.
Bacon conducted experiments into optics, although much of it was similar to what had been done and was being done at the time by Arab scholars. He did make a major contribution to the development of science in medieval Europe by writing to the Pope to encourage the study of natural science in university courses and compiling several volumes recording the state of scientific knowledge in many fields at the time. He described the possible construction of a telescope, but there is no strong evidence of his having made one. He recorded the manner in which he conducted his experiments in precise detail so that others could reproduce and independently test his results - a cornerstone of the scientific method, and a continuation of the work of researchers like Al Battani.
In the 14th century, some scholars, such as Jean Buridan and Nicole Oresme, started to question the received wisdom of Aristotle's mechanics. In particular, Buridan developed the theory of impetus, which was an important step towards the modern concept of inertia.
In his turn, Oresme showed that the reasons proposed by the physics of Aristotle against the movement of the earth were not valid and adduced the argument of simplicity for the theory that the earth moves, and not the heavens. In the whole of his argument in favour of the earth's motion Oresme is both more explicit and much clearer than that given two centuries later by Copernicus. He was also the first to assume that colour and light are of the same nature and the discoverer of the curvature of light through atmospheric refraction; even though, up to now, the credit for this latter achievement has been given to Hooke.
In the 14th century Europe was rocked by the Black Death which led to much social upheaval. In spite of this pause, the 15th century saw the artistic flourishing of the Renaissance. The rediscovery of ancient texts was improved when many Byzantine scholars had to seek refuge in the West after the fall of Constantinople in 1453. Meanwhile, the invention of printing was to make learning more accessible and allow a faster propagation of new ideas. All that paved the way to the Scientific Revolution, which may also be understood as a resumption of the process of scientific change halted around the middle of the 14th century.
Early modern physics
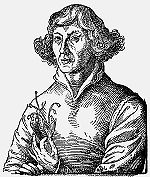
The early modern period is seen as a flowering of the Renaissance, in what is often known as the " Scientific Revolution", viewed as a foundation of modern science. Historians like Howard Margolis hold that the Scientific Revolution began in 1543, when Nicolaus Copernicus received the first copy of his De Revolutionibus, printed in Nuremberg (Nürnberg) by Johannes Petreius. Most of its contents had been written years prior, but the publication had been delayed. Copernicus died soon after receiving the copy.
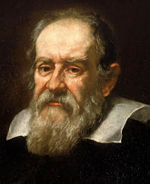
Further significant advances were made over the following century by Galileo Galilei, Christiaan Huygens, Johannes Kepler, and Blaise Pascal. During the early seventeenth century, Galileo made extensive use of experimentation to validate physical theories, which is the key idea in the modern scientific method. Galileo formulated and successfully tested several results in dynamics, in particular the Law of Inertia. In Galileo's Two New Sciences, a dialogue between the characters Simplicio and Salviati discuss the motion of a ship (as a moving frame) and how that ship's cargo is indifferent to its motion. Huygens used the motion of a boat along a Dutch canal to illustrate an early form of the conservation of momentum.
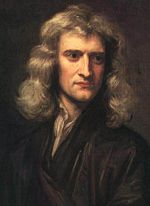
The scientific revolution is considered to have culminated with the publication of the Philosophiae Naturalis Principia Mathematica in 1687 by the mathematician, physicist, alchemist and inventor Sir Isaac Newton ( 1643- 1727). In 1687, Newton published the Principia, detailing two comprehensive and successful physical theories: Newton's laws of motion, from which arise classical mechanics; and Newton's law of universal gravitation, which describes the fundamental force of gravity. The Principia also included several theories in fluid dynamics.
From the late seventeenth century onward, thermodynamics was developed by physicist and chemist Boyle, Young, and many others. In 1733, Bernoulli used statistical arguments with classical mechanics to derive thermodynamic results, initiating the field of statistical mechanics. In 1798, Thompson demonstrated the conversion of mechanical work into heat, and in 1847 Joule stated the law of conservation of energy, in the form of heat as well as mechanical energy. Ludwig Boltzmann, in the nineteenth century, is responsible for the modern form of statistical mechanics.
Classical mechanics was re-formulated and extended by Leonhard Euler, French mathematician Joseph-Louis Comte de Lagrange, Irish mathematical physicist William Rowan Hamilton, and others, who produced new results in mathematical physics. The law of universal gravitation initiated the field of astrophysics, which describes astronomical phenomena using physical theories. Newton's Law of gravitation also helped put celestial mechanics on proper scientific and mathematical footing.
After Newton defined classical mechanics, the next great field of inquiry within physics was the nature of electricity. Observations in the seventeenth and eighteenth century by scientists such as Robert Boyle, Stephen Gray, and Benjamin Franklin created a foundation for later work. These observations also established our basic understanding of electrical charge and current.
By 1808 John Dalton had discovered that atoms of different elements have different weights and proposed the modern theory of the atom.
It was Hans Christian Ørsted who first proposed the connection between electricity and magnetism after observing the deflection of a compass needle by a nearby electric current. By the early 1830s Michael Faraday had demonstrated that magnetic fields and electricity could generate each other. In 1864 James Clerk Maxwell presented to the Royal Society a set of equations that described this relationship between electricity and magnetism. Maxwell's equations also predicted correctly that light is an electromagnetic wave.
The Scientific Revolution began in the late 16th century with only a few researchers, and evolved into an enterprise which continues to the present day. Starting with astronomy, the principles of natural philosophy] crystallized into fundamental laws of physics which were enunciated and improved in the succeeding centuries. By the 19th century, the sciences had segmented into multiple fields with specialized researchers and the field of physics, although logically pre-eminent, no longer could claim sole ownership of the entire field of scientific research.
16th century
In the 16th century Nicolaus Copernicus revived Aristarchus' heliocentric model of the solar system in Europe (which survived primarily in a passing mention in The Sand Reckoner of Archimedes). When this model was published at the end of his life, it was with a preface by Andreas Osiander that piously represented it as only a mathematical convenience for calculating the positions of planets, and not an account of the true nature of the planetary orbits.
In England William Gilbert (1544-1603) studied magnetism and electricity, and published a seminal work, De Magnete (1600), in which he thoroughly presented his numerous experimental results. Gilbert who designed the versorium: a device that detected the presence of statically charged objects.
17th century
In the early 17th century, the invention of the telescope and microscope, which is claimed to have been invented by three individuals ( Hans Lippershey, Jacob Metius, and Zacharias Jansen) would have profound implications on the history of science, in particular astronomy and physics. In the early 17th century Johannes Kepler formulated a model of the solar system based upon the five Platonic solids, in an attempt to explain why the orbits of the planets had the relative sizes they did. His access to extremely accurate astronomical observations by Tycho Brahe enabled him to determine that his model was inconsistent with the observed orbits. After a heroic seven-year effort to more accurately model the motion of the planet Mars (during which he laid the foundations of modern integral calculus) he concluded that the planets follow not circular orbits, but elliptical orbits with the Sun at one focus of the ellipse. This breakthrough overturned a millennium of dogma based on Ptolemy's idea of "perfect" circular orbits for the "perfect" heavenly bodies. Kepler then went on to formulate his three laws of planetary motion. He also proposed the first known model of planetary motion in which a force emanating from the Sun deflects the planets from their "natural" motion, causing them to follow curved orbits.
In 1643, Evangelista Torricelli invented the barometer, which arose from solving an important practical problem. Torricelli discovered Torricelli's Law, regarding the speed of a fluid flowing out of an opening, which was later shown to be a particular case of Bernoulli's principle. Torricelli also devised an equation sometimes called Torricelli's equation, which is used in the study of kinematics.
In 1660, Robert Hooke, an English scientist, formulated Hooke's law of elasticity, which describes the linear variation of tension with extension in an elastic spring.
An important device, the vernier, which allows the accurate mechanical measurement of angles and distances was invented by a Frenchman, Pierre Vernier, in 1631. It is in widespread use in scientific laboratories and machine shops to this day.
Otto von Guericke constructed the first air pump in 1650 and demonstrated the physics of the vacuum and atmospheric pressure using the Magdeburg hemispheres. Later, he turned his interests to static electricity, and he invented a mechanical device consisting of a sphere of sulfur that could be turned on a crank and repeatedly charged and discharged to produce electric sparks.
In 1656 the Dutch physicist and astronomer, Christian Huygens, invented a mechanical clock using a pendulum that swung through an elliptical arc, powered by a falling counterweight, to usher in the era of accurate timekeeping. Huygens also formulated Newton's second law of motion, but in quadratic form. Huygens greatest contribution comes from his early theory that light travels in waves (Wave–particle duality), and for his development of Huygens–Fresnel principle, along with French physicist Augustin-Jean Fresnel. This mathematical principle provided a method of analysis that could be applied to problems of wave propagation, and it would have applications in the later development of quantum mechanics.
The first quantitative estimate of the speed of light was made in 1676 by Ole Rømer, by timing the motions of Jupiter's satellite Io with a telescope.
During the early 17th century, Galileo Galilei made extensive use of experimentation to validate physical theories, which is the key idea in the scientific method. Galileo's use of experiment, and the insistence of Galileo and Kepler that observational results must always take precedence over theoretical results (in which they followed the precepts of Aristotle if not his practice), brushed away the acceptance of dogma, and gave birth to an era where scientific ideas were openly discussed and rigorously tested. Galileo formulated and successfully tested several results in dynamics, including the correct law of accelerated motion, the parabolic trajectory, the relativity of unaccelerated motion, and an early form of the Law of Inertia.
A French mathematician and scientist Blaise Pascal invented the hydraulic press, and an early calculator. Pascal also formulated Pascal's law, which states that for all points at the same absolute height in a connected body of an incompressible fluid at rest, the fluid pressure is the same, even if additional pressure is applied on the fluid at some place. Pascal also wrote many important papers on the scientific method.
René Descartes, French mathematician, philosopher, and natural scientist, invented analytic geometry, and discovered the law of conservation of momentum. He outlined his views on the universe in his Principles of Philosophy.
In 1687, Isaac Newton published the Philosophiae Naturalis Principia Mathematica, detailing two comprehensive and successful physical theories: Newton's laws of motion, from which arise classical mechanics; and Newton's Law of Gravitation, which describes the fundamental force of gravity. Newton's theory of gravity would be so successful that it would be used by William Herschel a century later to discover a new planet in the solar system, Uranus. The Law of Gravitation initiated the field of astrophysics and celestial mechanics, which describes astronomical phenomena using physical theories. In the Principia Mathematica Newton also enunciated the principles of conservation of momentum and the conservation of angular momentum. Later on in life Newton would move on to formulate the law of cooling and developed a theory of light based on his experiments with decomposing light through a prism. Newton would also invent a reflecting telescope and along with Gottfried Leibniz would move on to independently of one another invent calculus, which has many important applications in physics.
The 17th century would also witness the beginning of the metric system, which would result in the formation of set of standards for weight and measurements. Early work in developing the metric system were pioneered by John Wilkins, Gabriel Mouton, and Antoine Lavoisier among others.
18th century
From the 18th century onwards, thermodynamic concepts were developed by Robert Boyle, Thomas Young, and many others, concurrently with the development of the steam engine, onward into the next century. In 1733, Daniel Bernoulli used statistical arguments with classical mechanics to derive thermodynamic results, initiating the field of statistical mechanics. Bernoulli would also lay the foundation of kinetic molecular theory in 1738, with the publication of Hydrodynamica. In Hydrodynamica, Bernoulli would state that all gases consist of molecules that are constantly in motion, moving in all directions, and impacting on surfaces. It was these movements and impacts that resulted in pressure, and that heat is the kinetic energy of these moving molecules. Another pioneer of kinetic molecular theory during the 18th century was Mikhail Lomonosov. Kinetic molecular theory would have wide ranging applications in physics, in particular thermodynamics, and chemistry. Bernoulli would also move on to develop what become known as Bernoulli's principle. It states that when an ideal fluid that has no work acting on it, an increase in velocity will result in a simultaneous decrease in pressure or a change in the fluids gravitational potential energy. The principle plays a central role in fluid dynamics and would have considerable impact on the development of aerodynamics in the 19th and 20th century. During the beginning of the 18th century methods were slowly taking shape in order to provide a standard set of measures to determine temperature. One set of measure was put forward by Anders Celsius in 1742. A peculiarity of Celsius scale was that melting point of an object was set at 0 degrees Celsius and was not until a famous botanist, Carl Linnaeus, would have the scale inverted to read boiling point being at 100 degrees Celsius. Another method for determining temperature was put forward by Gabriel Fahrenheit, in 1724. Both methods are named after their respective originators and both are used interchangeably by scientists all over the world to the present date.
In 1729 the English Astronomer Royal James Bradley gave the first empirical proof of heliocentrism, by explaining stellar aberration from the hypotheses of the finite speed of light and the Earth annually orbiting the Sun, after which the prevailing geoheliocentric planetary models were abandoned in favour of heliocentrism.
In 1746 an important step in the development of electricity was taken in the invention of the Leyden jar, a capacitor, that could store and discharge electrical charge in a controlled way. In the 18th century many of the fundamental concepts about the nature of electricity were discovered. In 1733, C. F. du Fay, discovered the existence of two types of electricity and named them "vitreous" and "resinous" (later known as positive and negative charge respectively). William Watson, in 1747 discovered that a discharge of static electricity was equivalent to an electric current. Charles Augustin de Coulomb formulated Coulomb law, which gives the definition of the electrostatic force of attraction and repulsion. Nearing the 18th century André-Marie Ampère discovered the relationship that relates the circulating magnetic field in a closed loop to the electric current passing through the loop. Carl Friedrich Gauss would develop Gauss's law which is used in the electrostatic application of the generalized Gauss's theorem giving the equivalence relation between any flux, e.g. of liquids, electric or gravitational, flowing out of any closed surface and the result of inner sources and sinks, such as electric charges or masses enclosed within the closed surface. Nearing the end of the century the relationship that exists between magnetism and electricity continued to described which resulted in the formulation of the fundamental equation of the Biot-Savart Law, which is an equation in electromagnetism that describes the magnetic field vector B in terms of the magnitude and direction of the source electric current, the distance from the source electric current, and the magnetic permeability weighting factor. In the mid 18th century Henry Cavendish made important discoveries concerning electricity. Among Cavendish's discoveries were the concept of electric potential, an early unit of capacitance, a formula for the capacitance of a plate capacitor, concept of the dielectric constant of a material, and Laws for the division of current in parallel circuits, now attributed to Charles Wheatstone. Cavendish also devised a method that allowed for the first time in obtaining a numerical value for gravity. Benjamin Thompson]] demonstrated the conversion of unlimited mechanical work into heat. Other pioneers were Robert Boyle, who in 1675 stated that electric attraction and repulsion can act across a vacuum; Stephen Gray, who in 1729 classified materials as conductors and insulators and Luigi Galvani discovered that muscle and nerve cells produce electricity. Benjamin Franklin effectively used them (together with von Guericke's generator) in his researches into the nature of electricity in 1752 In about 1788, Joseph Louis Lagrange elaborated an important new formulation of mechanics using the calculus of variations, the principle of least action and the Euler–Lagrange equations This led to the development of what is called Lagrangian mechanics, which fuses classical mechanics with conservation of momentum and conservation of energy. The end of the 18th century would also witness a historic change as profound if not more than the agricultural revolution. It began with the conversion of steam into mechanical energy, which would ultimately lead to the industrial revolution. Various steam engines were developed from Thomas Newcomen's to James Watts steam engines. The industrial revolution is significant in terms of physics because as various steam engines were being developed there was a greater need to make them more powerful and efficient. It was this quest to make steam engines more efficient that would lead to the development of the branch of physics called thermodynamics ( History of thermodynamics). It is the subsequent discoveries in thermodynamics that led to technological wonders such as the internal combustion engine and other forms of heat engines, which are used to power airplanes, automobiles, locomotives, and all forms of mobile transport. The 18th century would witness an explosive growth of mathematical physics, which would help formulation of theories that would come about in the 19th and 20th century. The most important work mathematical physics came from the development of wave equations. These second-order linear partial differential equation that describes the propagation of a variety of waves, such as sound waves, light waves and water waves. They are extensively used fields such as acoustics, electro magnetics, and fluid dynamics. These equations were developed and studied by Jean le Rond d'Alembert, Leonhard Euler, Daniel Bernoulli, and Joseph-Louis Lagrange.
Modern physics
19th century
In a letter to the Royal Society in 1800, Alessandro Volta described his invention of the electric battery, thus providing for the first time the means to generate a constant electric current, and opening up a new field of physics for investigation. In announcing his discovery of the pile, Volta paid tribute to the influences of William Nicholson, Tiberius Cavallo and Abraham Bennet. Volta also studied what we now call capacitance, developing separate means to study both electrical potential V and charge Q, and discovering that for a given object they are proportional. This may be called Volta's Law of Capacitance, and likely for this work the unit of electrical potential has been named the volt.
The onset of 1800 also witnessed William Herschel's discoverey of infrared radiation by passing sunlight through a prism and holding a thermometer just beyond the red end of the visible spectrum. This thermometer was meant to be a control to measure the ambient air temperature in the room. He was shocked when it showed a higher temperature than the visible spectrum. Further experimentation led to Herschel's conclusion that there must be an invisible form of light beyond the visible spectrum.
Some of the most important experiments during the on set of the 19th century were performed by Thomas Young. Young would demonstrate the wave nature of light and finally provide evidence to overturn a long held theory put forward by Isaac Newton that light consisted of particles. With the ripple tank he demonstrated the idea of interference in the context of water waves. With the two-slit, or double-slit experiment, he demonstrated interference in the context of light as a wave. In the two-slit experiment, c. 1801, Young passed a beam of light through two parallel slits in an opaque screen; on the other side was a white screen, where a pattern of alternating light and dark bands formed. This supported Young's contention that light is composed of waves. Young performed and analyzed a number of experiments, including interference of light from reflection off nearby pairs of micrometer grooves, from reflection off thin films of soap and oil, and from Newton's rings. He also performed two important diffraction experiments using fibers and long narrow strips. Within ten years, much of Young's work was reproduced and then extended by Fresnel. In 1817, Young had proposed a small transverse component to light, while yet retaining a far larger longitudinal component. Fresnel, by the year 1821, was able to show via mathematical methods that polarization could be explained only if light was entirely transverse, with no longitudinal vibration whatsoever.
By 1808 John Dalton's earlier ideas about the existence of atoms of different weights and the law of multiple proportions had led him to the modern theory of the atom and many other theories used today in modern physics. The convergence of various estimates of Avogadro's number lent decisive evidence for atomic theory.
In 1807 Thomas Young described the characterization of elasticity that came to be known as Young's modulus, denoted as E, in 1807, and further described it in his subsequent works such as his 1845 Course of Lectures on Natural Philosophy and the Mechanical Arts, which is used to determine the stiffness of a material. In 1804 Young founded the theory of capillary phenomena on the principle of surface tension. He also observed the constancy of the angle of contact of a liquid surface with a solid, and showed how from these two principles to deduce the phenomena of capillary action.
In 1822, Claude-Louis Navier and George Gabriel Stokes would publish a series of papers on equations that are central to fluid dynamics and would later be called Navier–Stokes equations. These set of equations describe the motion of fluid substances such as liquids and gasses. The genius of these set of equations comes from their versatility in several varying branches of science. They can be used to model weather, ocean currents, water flow in pipes, blood flow, flow around an airfoil, motion of stars inside a galaxy, and when combined with Maxwell's equations can be used to model Magnetohydrodynamics. Therefore; Navier–Stokes equations play a central role design of aircraft, cars, power stations, and used in the analysis of the effects of pollution. Claude-Louis Navier would move onto to form the general theory of elasticity in 1821, and would eventually develop the elastic modulus in 1826. It is for his work on the elastic modulus that Navier is often referred to as the founder of structural analysis.
The Coriolis effect was discovered by Gaspard-Gustave Coriolis, in 1835. Coriolis effect is an apparent deflection of moving objects from a straight path when they are viewed from a rotating frame of reference. The mathematics used by Coriolis to describe this phenomenon appeared in the tidal equations of Pierre-Simon Laplace in 1778. The effect has a wide varitey of applications in physics but play a particularly important role in meteorology.
Although early investigations into a branch of physics called thermodynamics were done by Boyle, Hooke, and Guericke among others, the science did not come into its own until the work of a young French physicist and military engineer called Sadi Carnot. In 1824 Carnot published the Reflections on the Motive Power of Fire, which is considered the founding paper in thermodynamics. In this paper Carnot established a general theory of heat engines. Carnot outlined the principles of what would later become known as the Carnot cycle, the Carnot heat engine, Carnot theorem, and thermodynamic efficiency, to name a few. This monumental work laid the foundations for the first law of thermodynamics and the second law of thermodynamics.
The behaviour of electricity and magnetism was studied by Michael Faraday, Georg Ohm, Hans Christian Ørsted, and others. Faraday, who began his career in chemistry working under Humphry Davy at the Royal Institution, demonstrated that electrostatic phenomena, the action of the newly discovered electric pile or battery, electrochemical phenomena, and lightning were all different manifestations of electrical phenomena.. Faraday further discovered in 1821 that electricity can cause rotational mechanical motion, and in 1831 discovered the principle of electromagnetic induction, by which means a moving magnet induces an electrical current in a conductor. Thus it was Faraday who laid the foundations for both the electric motor and the electric generator. Faraday also formulated a physical conception of electromagnetic fields. Discoveries in electricity and magnetism would have a profound effect upon society. The principles of electromagnetism find applications in various allied disciplines such as microwaves, antennas, electric machines, satellite communications, bioelectromagnetics, plasmas, nuclear research, fibre optics, electromagnetic interference and compatibility, electromechanical energy conversion, radar meteorology, and remote sensing. Electromagnetic devices include transformers, electric relays, radio/TV, telephones, electric motors, transmission lines, waveguides, optical fibers, and lasers.
Classical mechanics was given a new formulation by William Rowan Hamilton ( Hamiltonian Mechanics), in 1833 with the introduction of what is now called the Hamiltonian, which a century later gave an entry to wave mechanical formulation of quantum mechanics. During its first 1868 meeting, notable oceanographer Matthew F. Maury helped launch the American Association for the Advancement of Science (AAAS) by giving a very influential lecture on Wind and Current Charts which gave rise to one of the first examples of international scientific collaboration.
James Clerk Maxwell built upon Michael Faraday's physical conception of electromagnetic fields with an interlinked set of twenty equations that explained the interactions between electric and magnetic fields and unified the two phenomena into a single theory of electromagnetism. Maxwell's equations were presented to the Royal Society in 1864. These twenty equations were later reduced, using vector calculus, to a set of four equations by Oliver Heaviside. A prediction of Maxwell's equations was that light is an electromagnetic wave. Confirmation of Maxwell's insight into electromagnetism was made with the observation, in 1888, and the discovery of radio by Heinrich Hertz and in 1895 when Wilhelm Roentgen detected X-rays. Aside from Maxwell's work in the electromagnetism, he would also make important contributions to thermodynamics through a set of equations called Maxwell Relations, which provide an experimental way in which to measure entropy. Maxwell along with Ludwig Boltzmann would establish Maxwell–Boltzmann, which is a probability distribution for molecular speeds in a gas.
In 1842, an Austrian scientist by the name of Christian Doppler would publish a paper on what would become known as the Doppler effect. The summation of his work was an equation which described a change in frequency and wavelength of a wave as perceived by an observer moving relative to the source of the waves. In 1848, John Scott Russell conducted a series of experiments to verify some of the conclusions of the Doppler effect. The equation has applications has applications in astronomy, temperature measurement, radar, medical imaging, flow measurement, and underwater acoustics.
Building upon the work of physicists before him a young German physicist by the name of Gustav Kirchhoff, while still a student in 1845, would formulate Kirchhoff's circuit laws, which are now used in all of electrical engineering. In 1859 Kirchoff would proceed to explain what became known as Kirchoff's law of thermal radiation, which provided a general statement about emission and absorption in heated objects. Later on in his career Kirchoff would work with Robert Bunsen to establish the field of spectroscopy, specifically through their formulation of Kirchoff's three laws of spectroscopy.
In 1847 James Prescott Joule stated the law of conservation of energy, in the form of heat as well as mechanical energy. However, the principle of conservation of energy had been suggested in various forms by perhaps a dozen German, French, British and other scientists during the first half of the 19th century. About the same time, entropy and the second law of thermodynamics were first clearly described in the work of Rudolf Clausius. In 1875 Ludwig Boltzmann made the important connection between the number of possible states that a system could occupy and its entropy. With two installments in 1876 and 1878, Josiah Willard Gibbs developed much of the theoretical formalism for thermodynamics, and a decade later firmly laid the foundation for statistical mechanics — much of which Ludwig Boltzmann had independently invented. In 1881 Gibbs also was very influential in moving much of the notation of physics from Hamilton's quaternions to vectors. From 1873-76 Gibbs would help to apply thermodynamics to chemical processes, thus laying the foundations of chemical thermodynamics. Gibbs would publish 3 papers, the most famous being titled On the Equilibrium of Heterogeneous Substances in which he demonstrated that thermodynamic processes could be graphically analyzed, by studying the energy, entropy, volume, temperature and pressure of the thermodynamic system, in such a manner to determine if a process would occur spontaneously. The first and second laws of thermodynamics emerged in the 1850s, primarily out of the works of William Rankine, Rudolf Clausius, and William Thomson. The third law of thermodynamics, which was established by Ludwig Boltzmann, states that the entropy of a pure substance approaches zero as the absolute temperature approaches zero.
The discovery of the Hall effect in 1879 gave the first direct evidence that the carrier of electricity was negatively charged. In 1879, Sir William Crookes would discover a new form of matter which he called "radiant matter". What Crookes has discovered was what became known as plasma, the most abundant state of matter in the universe. Crookes was able to make his discovery of plasma by inventing the Crookes tube. The discovery of plasma is important because it constitutes the first time since a new state of matter had been discovered, aside from common knowledge of solids, liquids, and gases. Secondly, further research into plasma demonstrated its importance not only to science but also many technological applications such as plasma displays, fusion energy research, and production of integrated circuits to name a few.
Dimensional analysis was used for the first time in 1878 by Lord Rayleigh who was trying to understand why the sky is blue. In 1887 the Michelson-Morley experiment was conducted and it was interpreted as counter to the generally held theory of the day, that the Earth was moving through a "luminiferous aether". Albert Abraham Michelson and Edward Morley were not fully convinced of the non-existence of the aether. Morley conducted further experiments with Dayton Miller with improved interferometers, again giving null results.
In 1887, Nikola Tesla investigated X-rays using his own devices as well as Crookes tubes. In 1895, Wilhelm Conrad Röntgen observed and analysed X-rays, which turned out to be high-frequency electromagnetic radiation. Radioactivity was discovered in 1896 by Henri Becquerel, and further studied by Pierre and Marie Curie and others. This initiated the field of nuclear physics.
In the late 19th century Johannes Diderik van der Waals would postulate the existence of forces which act between molecules, but are weak compared to those in chemical bonds. These forces would later be named Van der Waals forces. A German physict by the name of Fritz London would also discover similar forces which Waals had first proposed. Van der Waals forces occupy an important role in thermodynamics and chemistry.
In 1897, J. J. Thomson deduced that cathode rays were composed of negatively charged particles, which he called "corpuscles", later realized to be electrons. Philipp Lenard showed that the particles ejected in the photoelectric effect were the same as those in cathode rays, and that the energy of each particle was independent of the intensity of the light, but was greater for short wavelengths of the incident light.
These discoveries revealed that the assumption of many physicists, that atoms were the basic unit of matter, was flawed, and prompted further study into the structure of atoms, such as Ernest Rutherford's in 1911.
20th century
The turn of the 20th century brought the start of a revolution in physics. This revolution centered around the development of quantum mechanics and special and general relativity, the two concepts that mark the advent of modern physics. Both of these theories would offer fundamental insights into the universe and the laws the govern it. Quantum mechanics would describe the microscopic nature of matter, while relativity would describe space and time.
The zeroth law of thermodynamics, which is a generalized statement about bodies in contact at thermal equilibrium and the basis for the concept of temperature, was formalized in the early 20th century, although the principles behind the law were well known in the 19th century. In 1929, Lars Onsager, would establish Onsager reciprocal relations-sometimes referred to as the fourth law of thermodynamics.
The Lorentz transformations, the fundamental equations of special relativity, were published in 1897 and 1900 by Joseph Larmor and also in 1899 and 1904 by Hendrik Lorentz. They both showed that Maxwell's equations were invariant under the transformations. The ability to describe light in electromagnetic terms helped serve as a springboard for Albert Einstein's publication of the theory of special relativity in 1905. This theory combined classical mechanics with Maxwell's equations. The theory of special relativity unifies space and time into a single entity, spacetime. Relativity prescribes a different transformation between reference frames than classical mechanics; this necessitated the development of relativistic mechanics as a replacement for classical mechanics. In the regime of low (relative) velocities, the two theories agree.
In 1900, Max Planck published his explanation of blackbody radiation. This equation assumed that radiators are quantized, which proved to be the opening argument in the edifice that would become quantum mechanics. By introducing discrete energy levels, Planck, Einstein, Niels Bohr, and others developed quantum theories to explain other anomalous experimental results like the photoelectric effect. The discovery of quantum mechanics in the early 20th century revolutionized physics, and quantum mechanics is fundamental to most areas of current research.
In 1904, J. J. Thomson proposed the first model of the atom, known as the plum pudding model. In 1911, Ernest Rutherford deduced from scattering experiments the existence of a compact atomic nucleus, with positively charged constituents dubbed protons. The first quantum mechanical model of the atom, the Bohr model, was published in 1913 by Niels Bohr. Sir W. H. Bragg and his son Sir William Lawrence Bragg, also in 1913, began to unravel the arrangement of atoms in crystalline matter by the use of x-ray diffraction. Neutrons, the neutral nuclear constituents, were discovered in 1932 by James Chadwick.
One of the most important concepts in modern physics and one which would help usher in quantum mechanics was proposed by Louis de Broglie. Broglie's 1922 doctoral thesis, Recherches sur la théorie des quanta (Research on Quantum Theory), introduced his theory of electron waves. This included the wave-particle duality theory of matter, based on the work of Albert Einstein and Planck. This research culminated in the de Broglie hypothesis stating that any moving particle or object had an associated wave. De Broglie thus created a new field in physics, the mécanique ondulatoire, or wave mechanics, uniting the physics of light and matter. Among the applications of this work has been the development of electron microscopes to get much better image resolution than optical ones, because of shorter wavelengths of electrons compared with photons of visible light.
After his discovery of the atomic nucleus, Rutheford along with Frederick Soddy went on to achieve the first case of nuclear transmutation, a phenomenon which had been speculated since the time of the Greeks. Rutheford and Soddy noticed they had achieved this when, during their experiments in 1901, radioactive thorium converted into radium. The equivalence of mass and energy (Einstein, 1905) was spectacularly demonstrated during World War II, as research was conducted by each side into nuclear physics, for the purpose of creating a nuclear bomb. The German effort, led by Heisenberg, did not succeed, but the Allied Manhattan Project reached its goal. In America, a team led by Fermi achieved the first man-made nuclear chain reaction in 1942, and in 1945 the world's first nuclear explosive was detonated at Trinity site, near Alamogordo, New Mexico.
In 1915, Einstein extended special relativity to describe gravity with the general theory of relativity. One principal result of general relativity is the gravitational collapse into black holes, which was anticipated two centuries earlier, but elucidated by Robert Oppenheimer. Important exact solutions of Einstein's field equation were found by Karl Schwarzschild in 1915 and Roy Kerr only in 1963. One part of the theory of general relativity is Einstein's field equation. This describes how the stress-energy tensor creates curvature of spacetime and forms the basis of general relativity. Further work on Einstein's field equation produced results which predicted the Big Bang, black holes, and the expanding universe. Einstein believed in a static universe. He tried, and failed, to fix his equation to allow for this. In 1929, however, Edwin Hubble published his discovery that that the universe is expanding at a possibly exponential rate. This is the basis for understanding that the universe is expanding. Thus, the universe must have been smaller and therefore hotter in the past. In 1933 Karl Jansky at Bell Labs discovered the radio emission from the Milky Way, and thereby initiated the science of radio astronomy. By the 1940s, researchers like George Gamow proposed the Big Bang theory, evidence for which was discovered in 1964; Enrico Fermi and Fred Hoyle were among the doubters in the 1940s and 1950s. Hoyle had dubbed Gamow's theory the Big Bang in order to debunk it. Today, it is one of the principal results of cosmology.
According to Cornelius Lanczos, any physical law which can be expressed as a variational principle describes an expression which is self-adjoint or Hermitian. Thus such an expression describes an invariant under a Hermitian transformation. Felix Klein's Erlangen program attempted to identify such invariants under a group of transformations. Noether's theorem identified the conditions under which the Poincaré group of transformations (what is now called a gauge group) for general relativity define conservation laws. The relationship of these invariants (the symmetries under a group of transformations) and what are now called conserved currents, depends on a variational principle, or action principle. Noether's papers made the requirements for the conservation laws precise. Noether's theorem remains right in line with current developments in physics to this day.
In 1925 Wolfgang Pauli elucidated the Pauli exclusion principle and introduced the notion of quantized spin and fermions. Over the next several years, quantum mechanics would be formulated by Werner Heisenberg, Erwin Schrödinger and Paul Dirac in different ways which both explained the preceding heuristic quantum theories. In quantum mechanics, the outcomes of physical measurements are inherently probabilistic; the theory describes the calculation of these probabilities. It successfully describes the behaviour of matter at small distance scales. In 1925 Schrödinger formulated wave mechanics, which provided a consistent mathematical method for describing a wide variety of physical situations such as the particle in a box and the quantum harmonic oscillator which he solved for the first time. That same year Heisenberg described an alternative mathematical method, called matrix mechanics, which proved to be equivalent to wave mechanics. In 1928 Dirac produced a relativistic formulation built on Heisenberg's matrix mechanics, and predicted the existence of the positron and founded quantum electrodynamics. During the 1920s Schrödinger, Heisenberg, and Max Born were able to formulate a consistent picture of the chemical behaviour of matter, a complete theory of the electronic structure of the atom, as a byproduct of the quantum theory.
Quantum mechanics also provided the theoretical tools for condensed matter physics, whose largest branch is solid state physics. It studies the physical behaviour of solids and liquids, including phenomena such as crystal structures, semiconductivity, and superconductivity. The pioneers of condensed matter physics include Felix Bloch, who created a quantum mechanical description of the behaviour of electrons in crystal structures in 1928. Much of the behaviour of solids was elucidated within a few years with the discovery of the Fermi surface which was based on the idea of the Pauli exclusion principle applied to systems having many electrons. The understanding of the transport properties in semiconductors as described in William Shockley's Electrons and holes in semiconductors, with applications to transistor electronics enabled the electronic revolution of the twentieth century through the development of the ubiquitous, ultra-cheap transistor. The transistor was developed by physicists John Bardeen, Walter Houser Brattain, and William Bradford Shockley in 1947 at Bell Laboratories. The transistor is a semiconductor device that can be used to amplify or switch electronic signals. The transistor is the fundamental building block of computers, and all other modern electronic devices. The invention of the transistor and the integrated circuit would usher in the electronics age and the subsequent information revolution.
During the early stages of particle physics, physicists noticed a type of radioactive decay that occurred in the atomic nucleus, which gave of alpha particles that consisted of two protons and neutrons in a particle that resembled the helium nucleus. The phenomenon would be called alpha decay. In 1928, George Gamow used the concept of quantum tunneling, formulated earlier in quantum mechanics, to successfully explain alpha decay. Quantum tunneling states there is a process by which a particles can penetrate a potential barrier that has a higher kinetic energy then the particle itself. The principle of quantum tunneling would have important implications for all of particle physics, and as time passed would have practical technological applications.
In 1934, the Italian physicist Enrico Fermi had discovered strange results when bombarding uranium with neutrons, which he believed at first to have created transuranic elements. In 1939, it was discovered by the chemist Otto Hahn and the physicist Lise Meitner that what was actually happening was the process of nuclear fission, whereby the nucleus of uranium was actually being split into two pieces, releasing a considerable amount of energy in the process. At this point it became clear to a number of scientists independently that this process could potentially be harnessed to produce massive amount of energy, either as a civilian power source or as a weapon. Leó Szilárd actually filed a patent on the idea of a nuclear chain reaction in 1934. In America, a team led by Fermi and Szilárd achieved the first man-made nuclear chain reaction in 1942 in the world's first nuclear reactor, and in 1945 the world's first nuclear explosive was detonated at Trinity Site, north of Alamogordo, New Mexico. After the war, central governments would become the primary sponsors of physics. The scientific leader of the Allied project, theoretical physicist Robert Oppenheimer, noted the change of the imagined role of the physicist when he noted in a speech that:
- "In some sort of crude sense, which no vulgarity, no humor, no overstatement can quite extinguish, the physicists have known sin, and this is a knowledge which they cannot lose."
Though the process had begun with the invention of the cyclotron by Ernest O. Lawrence in the 1930s, nuclear physics in the postwar period entered into a phase of what historians have called " Big Science", requiring costly huge accelerators and particle detectors, and large collaborative laboratories to test open new frontiers. The primary patron of physics became central governments, who recognized that the support of "basic" research could sometimes lead to technologies useful to both military and industrial applications. Toward the end of the twentieth century, a European collaboration of 20 nations, CERN, became the largest particle physics laboratory in the world.
Another "big science" was the science of ionized gases, plasma, which had begun with Crookes tubes late in the 19th century. Large international collaborations over the last half of the twentieth century embarked on a long range effort to produce commercial amounts of electricity through fusion power, which remains a distant goal.
Further understanding of the physics of metals, semiconductors and insulators led a team of three men at Bell labs, William Shockley, Walter Brattain and John Bardeen in 1947 to the first transistor and then to many important variations, especially the bipolar junction transistor. Further developments in the fabrication and miniaturization of integrated circuits in the years to come produced fast, compact computers that came to revolutionize the way physics was done—simulations and complex mathematical calculations became possible that were undreamed of even a few decades previous.
The discovery of nuclear magnetic resonance in 1946 led to many new methods for examining the structures of molecules and became a very widely used tool in analytical chemistry, and it gave rise to an important medical imaging technique, magnetic resonance imaging.
Quantum field theory was formulated in order to extend quantum mechanics to be consistent with special relativity, and was developed in the late 1940s in the work of Richard Feynman, Julian Schwinger, Sin-Itiro Tomonaga, and Freeman Dyson. They formulated the theory of quantum electrodynamics, which describes the electromagnetic interaction, and successfully explained the Lamb shift. This provided the framework for modern particle physics, which studies fundamental forces and elementary particles. Chen Ning Yang and Tsung-Dao Lee, in the 1950s, discovered an unexpected asymmetry in the decay of a subatomic particle. In 1954, Yang and Robert Mills then developed a class of gauge theories which provided the framework for understanding the nuclear forces (Yang, Mills 1954). The theory for the strong nuclear force was first proposed by Murray Gell-Mann. The electroweak force, the unification of the weak nuclear force with electromagnetism, was proposed by Sheldon Lee Glashow, Abdus Salam, and Steven Weinberg and confirmed in 1964 by James Watson Cronin and Val Fitch. This led to the so-called Standard Model of particle physics in the 1970s, which successfully describes all the elementary particles observed to date.
The invention of bubble chambers and spark chambers in the 1950s allowed physicists to discover large amounts of particles called hadrons ( Timeline of particle discoveries). There needed to exist some way of classifying these sub-atomic particles and how they interact with the fundamental forces in nature. This led physicists such as Gell-Mann, George Zweig, and others to development of Quantum chromodynamics, which describes the interaction between quarks and gluons found in hadrons. Two interesting properties of this theory are asymptotic freedom, and confinement. Asymptotic freedom was later discovered in 1973 by David Politzer, Frank Wilczek, and David Gross.
Starting in 1960 the military establishment of the United States began using atomic clocks to construct the global positioning system which in 1984 achieved its full configuration of 24 satellites in low earth orbits. This came to have many important civilian and scientific uses as well. Investigations into quantum electronics by Charles Hard Townes, Nikolay Basov, Aleksandr Prokhorov, and Theodore Maiman in 1960's resulted in the development of lasers. When lasers were invented, they were called "a solution looking for a problem". Since then, they have become ubiquitous, finding utility in thousands of highly varied applications in every section of modern society, including consumer electronics, information technology, science, medicine, industry, law enforcement, entertainment, and the military. Superconductivity, discovered in 1911 by Kamerlingh Onnes, was shown to be a quantum effect and was satisfactorily explained in 1957 by Bardeen, Cooper, and Schrieffer. A family of high temperature superconductors, based on cuprate perovskite, were discovered in 1986, and their understanding remains one of the major outstanding challenges for condensed matter theorists.
In 1974 Stephen Hawking discovered the spectrum of radiation emanating during the collapse of matter into black holes. These mysterious objects became of intense interest to astrophysicists and even the general public in the latter part of the twentieth century.
The concept of quantum tunneling would be used to create a Scanning tunneling microscope in 1981 by Gerd Binnig and Heinrich Rohrer. The invention of the atomic force microscope (AFM) Scanning Tunneling Microscope (STM) are two early versions of scanning probes that launched nanotechnology. Scanning probe microscopy is an important technique both for characterization and synthesis of nanomaterials. Atomic force microscopes and scanning tunneling microscopes can be used to look at surfaces and to move atoms around. By designing different tips for these microscopes, they can be used for carving out structures on surfaces and to help guide self-assembling structures. By using, for example, feature-oriented scanning- positioning approach, atoms can be moved around on a surface with scanning probe microscopy techniques. At present, it is expensive and time-consuming for mass production but very suitable for laboratory experimentation.
The independent discovery of a quantum mechanical effect, giant magnetoresistance, in 1988 by Albert Fert and Peter Grünberg is considered as the birth of spintronics. The effect manifests itself as a significant decrease in electrical resistance in the presence of a magnetic field. In the absence of an applied magnetic field the direction of magnetization of adjacent ferromagnetic layers is antiparallel due to a weak anti-ferromagnetic coupling between layers, and it decreases to a lower level of resistance when the magnetization of the adjacent layers align due to an applied external field. The spins of the electrons of the nonmagnetic metal align parallel or antiparallel with an applied magnetic field in equal numbers, and therefore suffer less magnetic scattering when the magnetizations of the ferromagnetic layers are parallel. The effect is exploited commercially by manufacturers of hard disk drives.
The two themes of the twentieth century, general relativity and quantum mechanics, appear inconsistent with each other. General relativity describes the universe on the scale of planets and solar systems, while quantum mechanics operates on sub-atomic scales. This challenge is being attacked by string theory, which treats spacetime as composed, not of points, but of one-dimensional objects, strings. Strings have properties similar to a common string (e.g., tension and vibration). The theories yield promising, but not yet testable, results. The search for experimental verification of string theory is in progress.
Attempts to unify quantum mechanics and general relativity made significant progress during the 1990s. At the close of the century, a Theory of everything was still not in hand, but some of its characteristics were taking shape. String theory, loop quantum gravity and black hole thermodynamics all predicted quantized spacetime on the Planck scale.
A number of new efforts to understand the physical world arose in the last half of the twentieth century that generated widespread interest: fractals and scaling, self-organized criticality, complexity and chaos, power laws and noise, networks, non-equilibrium thermodynamics, sandpiles, nanotechnology, cellular automata and the anthropic principle were only a few of these important topics.
Future directions

Research in physics is progressing constantly on a large number of fronts, and is likely to do so for the foreseeable future.
In condensed matter physics, the greatest unsolved theoretical problem is the explanation for high-temperature superconductivity. Strong efforts, largely experimental, are being put into making workable spintronics and quantum computers.
In particle physics, the first pieces of experimental evidence for physics beyond the Standard Model have begun to appear. Foremost amongst these are indications that neutrinos have non-zero mass. These experimental results appear to have solved the long-standing solar neutrino problem in solar physics. The physics of massive neutrinos is currently an area of active theoretical and experimental research. In the next several years, particle accelerators will begin probing energy scales in the TeV range, in which experimentalists are hoping to find evidence for the Higgs boson and supersymmetric particles.
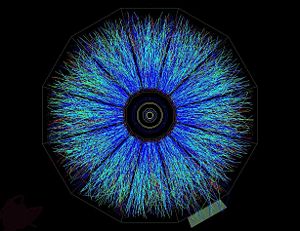
Theoretical attempts to unify quantum mechanics and general relativity into a single theory of quantum gravity, a program ongoing for over half a century, have not yet borne fruit. Currently, the leading candidates are M-theory, superstring theory, and loop quantum gravity.
Many astronomical and cosmological phenomena have yet to be explained satisfactorily, including the existence of ultra-high energy cosmic rays, the baryon asymmetry, the nature of non-baryonic dark matter, and the acceleration of the universe.
Although much progress has been made in high-energy, quantum, and astronomical physics, many everyday phenomena, involving complexity, chaos, or turbulence remain poorly understood. Complex problems that would appear to be soluble by a clever application of dynamics and mechanics, such as the formation of sand piles, nodes in trickling water, the shape of water droplets, mechanisms of surface tension catastrophes, or self-sorting in shaken heterogeneous collections are unsolved.
These complex phenomena have received growing attention since the 1970s for several reasons, not least of which has been the availability of modern mathematical methods and computers, which enabled complex systems to be modeled in new ways. The interdisciplinary relevance of complex physics also has increased, as exemplified by the study of turbulence in aerodynamics, or the observation of pattern formation in biological systems. In 1932, Horace Lamb correctly prophesied the success of the theory of quantum electrodynamics and the near-stagnant progress in the study of turbulence:
"I am an old man now, and when I die and go to heaven there are two matters on which I hope for enlightenment. One is quantum electrodynamics, and the other is the turbulent motion of fluids. And about the former I am rather optimistic."